Program Focus
Our research is centered on methanogenic archaea ("methanogens"), ancient microorganisms that comprise a large and diverse group of organisms within the archaeal domain of life. Their sole source of energy is methanogenesis, a form of anaerobic respiration that reduces simple oxidized carbon compounds to generate methane as an end product. Methanogenesis generates over a billion tons of methane each year, which accounts for at least 70% of global methane emissions. Given that methane is a potent greenhouse gas as well as a useful energy source, understanding methanogenic metabolism is an important endeavor that could lead to methane mitigation strategies and support bioenergy technologies. Methanogens contain a wealth of unusual biochemistry, much of which remains to be discovered or fully understood. My lab seeks to uncover and characterize novel enzymes, reactions, and biomolecules in methanogens. We are specifically interested in radical SAM enzymes in methanogens as well as the role of coenzyme F430 modifications in the methyl-coenzyme M reductase reaction.
Current Projects
Determining the functions and biosynthesis of coenzyme F430 modifications
Coenzyme F430 is a nickel-containing tetrapyrrole that is required for the final step of methanogenesis and the first step of anaerobic methane oxidation catalyzed by methyl-coenzyme M reductase (MCR). We recently discovered two modified versions of coenzyme F430 in methanogens - the structures of which we propose are mercaptopropionate-F430 (mp-F430) and mercaptopropanamide-F430 (mpa-F430). We propose that F430 modifications are involved in fine-tuning the MCR active site to enhance catalytic efficiency, influence dynamics and stability, and/or to guide the directionality of the MCR reaction. Current research is focused on demonstrating the functions of F430 modifications using genetic, physiological, and biochemical studies. We are also investigating the enzymes involved in the biosynthesis of F430 modifications.
Compatible solute biosynthesis
In order to cope with salt stress in high salinity environments, organisms must accumulate ions or organic compounds inside the cell in order to keep water from leaving the cell via osmosis. The organic compounds used for this function are known as osmolytes or compatible solutes. The major compatible solutes in methanogens are N-acetyl-beta-lysine and beta-glutamate, both of which require a radical S-adenosyl-L-methionine (SAM) enzyme for their biosynthesis. Radical SAM enzymes catalyze diverse and complicated radical-mediated chemistry using a [4Fe-4S] cluster and SAM. We are currently working to understand the physiological importance and enzymology of radical SAM aminomutases in methanogens.
Methylated pterin biosynthesis
Methanopterin is a one-carbon (C1) carrier coenzyme present in methanogens that is structurally and functionally similar to folate, the more common C1 transfer coenzyme. One difference in the structure of methanopterin compared to folate is the presence of two methyl groups at the C-7 and C-9 positions of the pterin. We have identified a radical SAM enzyme that catalyzes the addition of both methyl groups to a pterin-containing precursor. Our current work is focused on uncovering the mechanism of action of this enzyme as well as the physiological importance of methyl groups on methanopterin.
Self-sacrificing pABA synthases
Following our interests in cofactor biosynthesis pathways, we have expanded our work outside of methanogens to apply our expertise in metalloenzymes to understand a novel pathway for folate biosynthesis that involves a self-sacrificing enzyme required for the synthesis of p-aminobenzoate (pABA). We are investigating the mechanism of these novel enzymes, which use a dimetal cofactor and oxygen to cleave an active site tyrosine residue from the backbone to be used as the precursor to pABA.
Funding
We are grateful to the NSF (NSF-CHE 2105598) and DOE (DE-SC0022338) for funding our research.
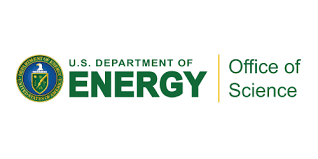
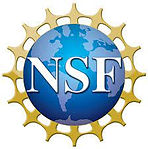